Towards probing the diffuse supernova neutrino background in all flavors
Core-collapse supernovae are among the most spectacular and efficient neutrino factories known so far. Detection of these neutrinos gives us an incredible opportunity to probe physics in extreme conditions not accessible on Earth. But so far, even with the detection of about twenty electron antineutrinos from SN 1987 A, we are yet to test even the most basic prediction about the neutrino emission, i.e., different neutrino flavors produced during the collapse share a comparable amount of the released energy. Existing detectors can register significantly more neutrinos of all flavors from the closeby core-collapse supernova and test this assumption. Unfortunately, this extreme phenomenon rarely occurs in our galaxy and its vicinity, only a few times per century.
What is more, while the multichannel detection of the next nearby supernova undoubtedly will allow us to measure and infer certain pieces of information, these will be solely about a single star. Theoretically, we expect a lot of common features, but nature may surprise us. Because of that, it is essential to observe neutrinos from multiple supernova events. Instead of waiting for centuries to get them, a better way of obtaining these is to build a sensitive enough detector capable of observing neutrinos from all the past collapses in our Universe – the diffuse supernova neutrino background (DSNB).
The dream of having such detectors might be finally coming true. The Super-Kamiokande (SK) detector achieved the most stringent upper limit on the electron antineutrino component of the DSNB. This limit is only a factor of 2-3 above most of the theoretical predictions. In addition, SK is now enriched with gadolinium which will help to reduce backgrounds for the DSNB search and most probably lead to the detection within near future.
The electron neutrino component of the DSNB has a ten times weaker upper limit than the electron antineutrino component. But with the upcoming Deep Underground Neutrino Experiment (DUNE), the limit may change into observation. As impressive as it sounds, capturing the complete picture of the core-collapse supernova landscape and investigating new astrophysics or physics requires probing DSNB in all flavors. But the upper limits on the non-electron component of the DSNB (muon and tau neutrinos and antineutrinos) are approximately thousand times weaker than the theoretical predictions. New ideas are needed to improve DSNB sensitivity to non-electron flavors.
In this work, we show that large-scale direct dark matter detectors can help significantly tighten the upper limits on the non-electron component of DSNB. The primary goal of these detectors is to search for dark matter, which means that they have low backgrounds and can also observe very feeble interactions. Both of these features are great for detecting only weakly interacting neutrinos. In addition, the neutrino detection channel in the direct dark matter detectors is equally sensitive to all neutrino flavors, which means that it is excellent for detecting the non-electron component of the DSNB.
We find that the one of the presently running detectors — XENONnT — can improve the current limit by a factor of three, while the next generation experiments, like for example DARWIN, up to a hundred times, bringing it almost to the level of the limit on the electron antineutrino component of the DSNB. We also show that the proposed lead-based supernova detectors RES-NOVA may improve on that. While it is not yet a detection perspective, this could shut the door for some non-standard scenarios that affect non-electron flavors and pave the way towards detection.
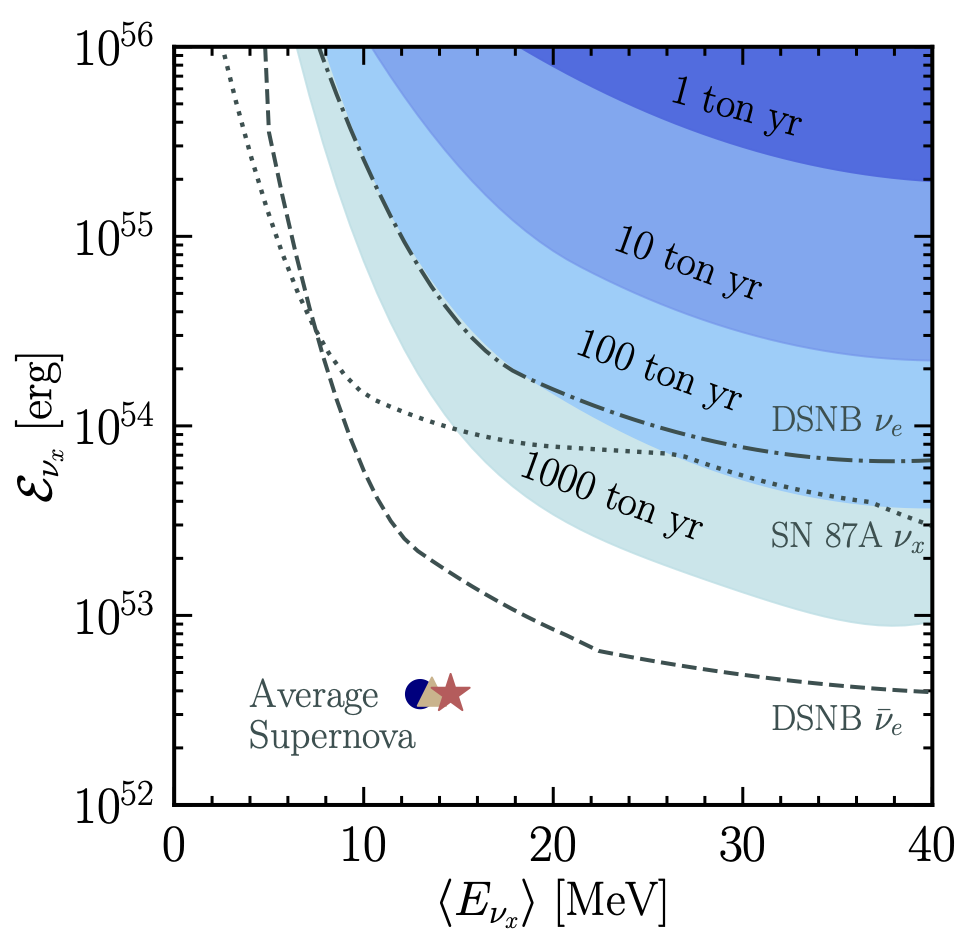
This introduction was prepared by N3AS Fellow Anna M. Suliga. Header image via kurzgesagt.org.
Read More: