Signatures of the quark-hadron phase transition at neutrino detectors
Prepared by Anna M. Suliga
Core-collapse supernovae are one of the most complex phenomena and most efficient neutrino factories in the Universe. Not only are they one of the sites of the production of the heavy elements which enable the existence of life, but they are also one of the most extreme environments we can indirectly probe.
The centers of the core-collapse supernovae are one of the densest, hottest, and most degenerate environments in the Universe. In such extreme conditions, the matter may no longer be in the same form as we typically observe on Earth, where the fundamental blocks of matter are quarks bound in nucleons. Instead, in the supernova cores, the matter may undergo a phase transition to pure quark matter. The abrupt change in the pressure caused by the transition can drive a supernova explosion and may imprint a sharp signature in the neutrino signal. In our work, we show how detecting such a feature at large-scale neutrino detectors can set competitive limits on the neutrino masses and help to localize the star.
Neutrinos are the first messengers we can observe from the core-collapse supernova because they interact only weakly. Due to their frequent interactions with the star’s envelope, photons ooze out slowly with an hour-to-day delay compared to neutrinos. Any prompt information about the supernova direction represents a valuable tool to alert astronomers and allow for a rapid multimessenger follow-up.
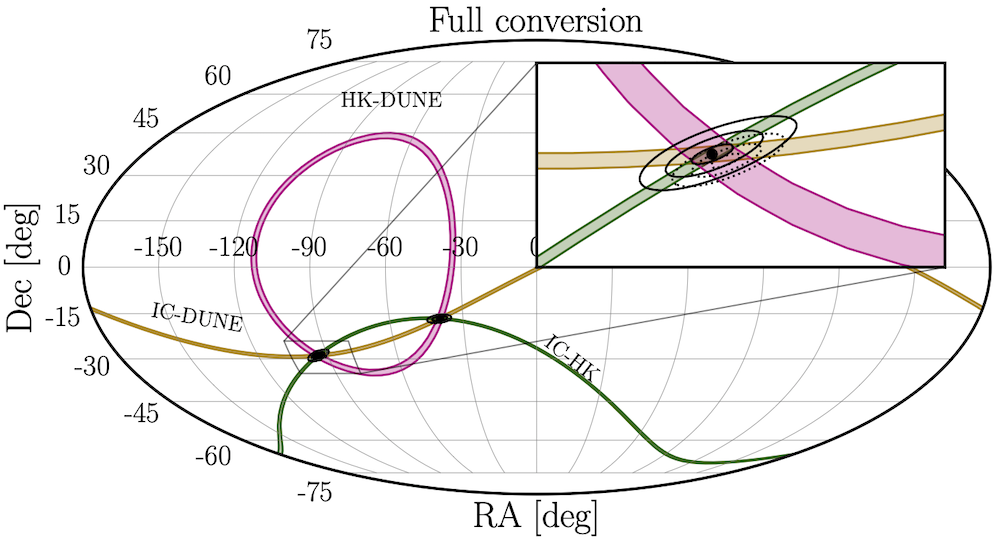
One of the methods to localize the supernova is triangulating the neutrino signal, which is based on measuring the arrival time delay between pairs of widely separated detectors around the globe. Each pair of detectors narrows down the location of the supernova to a cone in the sky. The width of the cone is determined by the uncertainty in measuring the onset of the sharp feature in the neutrino signal.
In our work, we show that triangulating supernova by detecting the quark-hadron phase transition peak can significantly, up to an approximate factor of 10, improve the pointing precision of the supernova localization within the galactic neighborhood compared to using the neutronization peak – a broader peak present in neutrino signal from supernova without invoking the phase transition to quark matter. Figure 1 shows the localization precision obtained by using three existing and upcoming large-scale neutrino detectors, Ice Cube (IC), Hyper-Kamiokande (HK), and DUNE, for the phase transition neutrino burst at a distance ten kpc from the Earth assuming full flavor swap between different neutrino flavors.
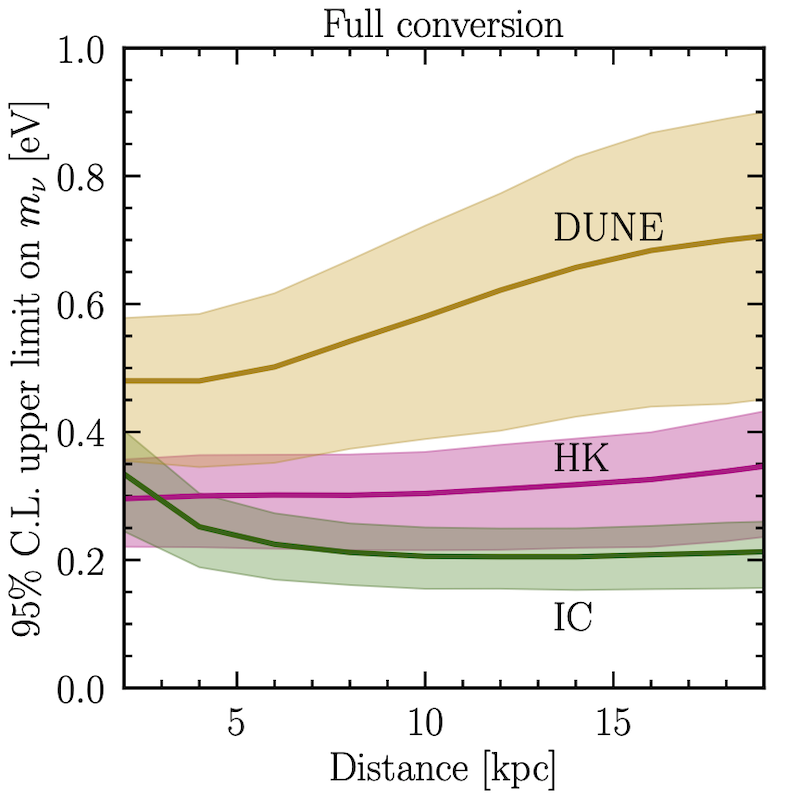
Any sharp feature in the supernova neutrino signal may also constrain the absolute neutrino mass because massive neutrinos introduce distinct energy-dependent time delays. The nonzero neutrino mass causes low-energy neutrinos to arrive later than high-energy ones. Therefore, the sharper the expected feature, the better the mass limit because more massive neutrinos smear the peak out onward later times. We find that IC can reach the 95% C.L. sensitivity of 0.16 eV, HK 0.22 eV, and DUNE 0.58 eV for supernova at a distance ten kpc from the Earth for favorable conversion scenarios, i.e., no neutrino conversions for HK and IC, and full flavor swap for DUNE. Figure 2 shows the change in the calculated mass limit in the three considered detectors with the distance to the supernova in a full-flavor swap neutrino conversion scenario.
Header photo: A side-by-side comparison showing observations of the Southern Ring Nebula in near-infrared light, at left, and mid-infrared light, at right, from NASA’s Webb Telescope. Credit: NASA and the Space Telescope Science Institute (STScI)
Read More: