Quantifying Nuclear Structure Uncertainties in Dark Matter Direct Detection Experiments
Prepared by Daniel Heimsoth and Brandon Lem
Dark matter is one of the least understood phenomena in modern physics. Despite making up the majority of the matter in the observable universe, its apparently weak interaction with ordinary matter makes it difficult to detect and study.
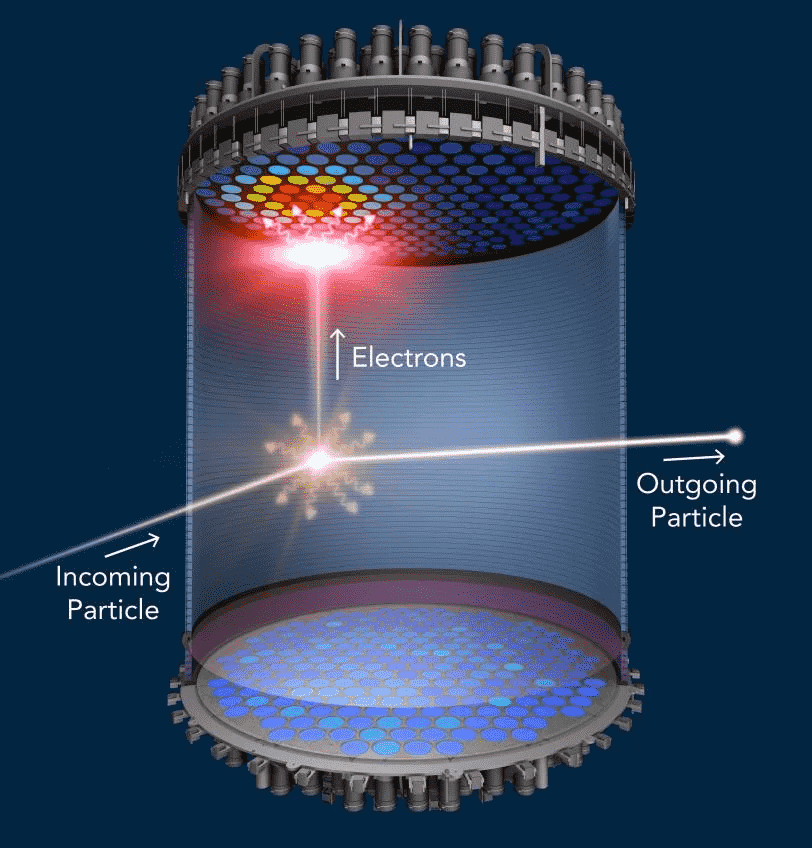
Credit: SLAC National Accelerator Laboratory
One method researchers use to search for dark matter is to directly look for non-gravitational interactions between dark matter and Standard Model particles in large detectors. Current and future dark matter direct detection experiments seek interaction events, though so far no positive dark matter particle interactions have been discovered. This allows us to place limits on the interaction cross sections and coupling coefficients which quantify how strongly dark matter and ordinary matter could couple to each other through different interaction channels. Because direct detection experiments require such a high level of sensitivity to detect dark matter, any potential errors and uncertainties may significantly alter the results produced by the experiment.
An often-neglected source of uncertainty in heavy-isotope-based detectors such as XENONnT and LUX-ZEPLIN (which both use liquid xenon as the target medium for interactions) is from modeling the nuclear structure and dark matter’s interactions with these complicated nuclei. These experiments are used to look for a possible class of dark matter known as weakly interacting massive particles (WIMPs); for WIMP masses greater than 10 GeV/c2, the dark matter can be considered non-relativistic. This allows researchers to focus on the low-energy theory of dark matter-nucleus interactions, which can be decomposed into fifteen so-called effective field theory (EFT) operators. This approach was developed for common dark matter direct detection interactions by Wick Haxton and collaborators in Fitzpatrick et al. (2013).
In our work, we carried out a preliminary theoretical analysis of the potential error in direct detection results due to uncertainties in nuclear structure. Considering two different nuclear structure models, Gaussian distributions were calculated for each nuclear density matrix element, which in turn defines nuclear response functions. We ran a Monte Carlo simulation, drawing random values for the matrix elements from the Gaussian distributions and passing in these generated matrices to dmscatter, an effective field theory dark WIMP-nucleus interaction code. For each iteration, we calculated the ideal event rates expected in the XENON1T detector for different WIMP masses and operator coupling strengths. By including a realistic modeling of the detector, we also found the minimal coupling coefficient values needed for a positive detection, along with their uncertainties derived from the nuclear structure modeling.
We found that certain low-energy coupling coefficients could have uncertainties above 50% due to nuclear modeling, particularly those that couple the nuclear spin to dark matter (see Figure 1). Crucially, different coupling operator coefficient uncertainties responded differently to changing WIMP mass. As more experiments consider the full EFT approach as we did instead of the simpler spin-independent vs. spin-dependent interactions, recognizing all sources of error in each coupling channel will become critical to set accurate limits and increase precision on WIMP properties through direct detection experiments.
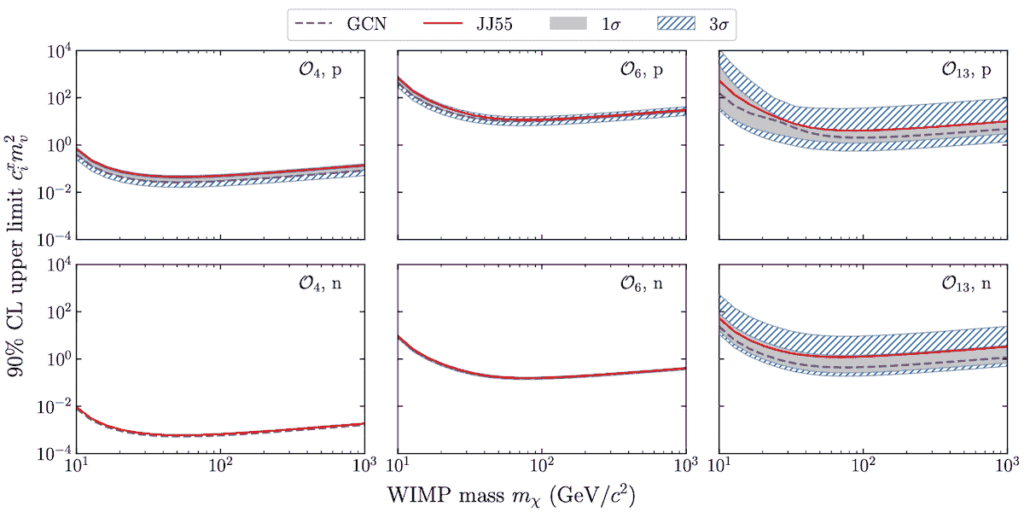
Read More: