Electromagnetic Signatures from the Tidal Tail of a Black Hole – Neutron Star Merger
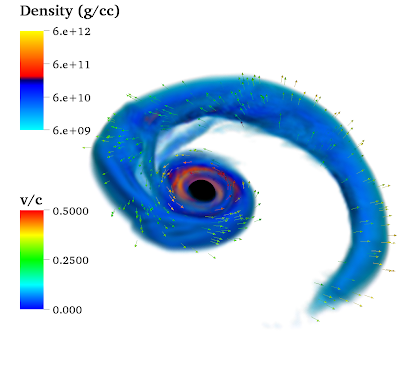
Black hole – neutron star (BH-NS) mergers are a major target for ground-based gravitational wave (GW) observatories. In its third observing run, the GW detector network (advance LIGO, Virgo, KAGRA) observed the first two candidate BH-NS coalescences: GW200105 and GW200115.
BH-NS mergers may eject neutron-rich matter that assembles into heavy elements through rapid neutron capture (i.e. the r-process), powering an electromagnetic (EM) transient known as a kilonova. Optical and infrared observations of kilonovae allow us to directly probe r-process material at its production site, providing an opportunity to address the long standing question of the origin of the heaviest elements. However, detailed computational models are needed to accurately infer parameters the ejecta parameters (e.g., mass, composition) from the light curve and spectral observations.
In recent work, our group (N3AS collaborators from UC Berkeley and the University of New Hampshire) studied the long-term evolution of the unbound dynamical ejecta of a BH-NS merger and how the resulting kilonova emission depended on viewing angle. We considered the ejecta from a BH-NS binary with an initial NS mass , BH mass
, dimensionless BH spin
, and BH spin inclination
. The data was obtained from a numerical relativity (NR) simulation at
ms after merger. The unbound component at this time has a mass
and characteristic velocity
.
NR simulations are the main tool to study dynamics in the strong gravity regime, but they are very intensive and can only be extended to a few milliseconds after the merger. We therefore tracked the post-merger evolution using general relativistic hydrodynamic simulations in which the BH gravity was treated as a fixed background spacetime. We included heating from the r-process nuclear reactions, which will modify the ejecta pressure and temperature. The heating rate was modeled using a parameterized function derived from detailed nuclear reaction networks. We ran the simulation to s, at which time the matter is effectively in free, homologous expansion.
Initially, the ejecta from the merger had the shape of a flattened spiral arc in the xy-plane. The r-process heating, however, inflated the matter in the direction perpendicular to the ejecta plane, making the ejecta more spherical. It also smoothed out the small-scale inhomogeneities and produced a larger spread in the velocity distribution (see Figure 1).
We then calculated the EM signatures of the ejecta using a separate Monte Carlo radiative transfer (MCRT) code. The photon-matter interactions are dominated by the atomic lines of the newly-formed neutron-rich nuclei, which we modeled using a calibrated analytic opacity function. We found that the the observable kilonova light curves varied with viewing angle due to the interplay of two effects: (1) parallel projected area: the light curves are brighter at viewing angles that perceive larger projected areas of the ejecta; and (2) Doppler enhancement/reduction: the luminosity is larger in viewing directions more closely aligned to the bulk motion of the ejecta. The peak bolometric luminosity varied by up to a factor of 3 with viewing angle (see Figure 2). The variations in the optical band light curves were even more dramatic, due to the Doppler modification shifting infrared light into or out of the visible bands depending on the viewing angles. The V-band peak magnitude reached
mag in the direction of bulk motion, suggesting brighter optical emission than suggested by some previous one and two-dimensional models. These results will help to interpret kilonova observations from BH-NS mergers and inform search strategies.
![Two charts showing z/10^11 [cm] versus y/10^11 [cm]](https://n3as.berkeley.edu/wp-content/uploads/2021/09/fig1-blackhole-1024x430.jpg)
![Figure 2, chart of colored lines curving up and then descending. Bolometric Luminosity vs t [d]](https://n3as.berkeley.edu/wp-content/uploads/2021/09/fig2-merger-1024x678.jpg)


This introduction was prepared by Siva Darbha, UC Berkeley graduate student.
Read More: