Neutron Star Wars: The Strong Force Awakens
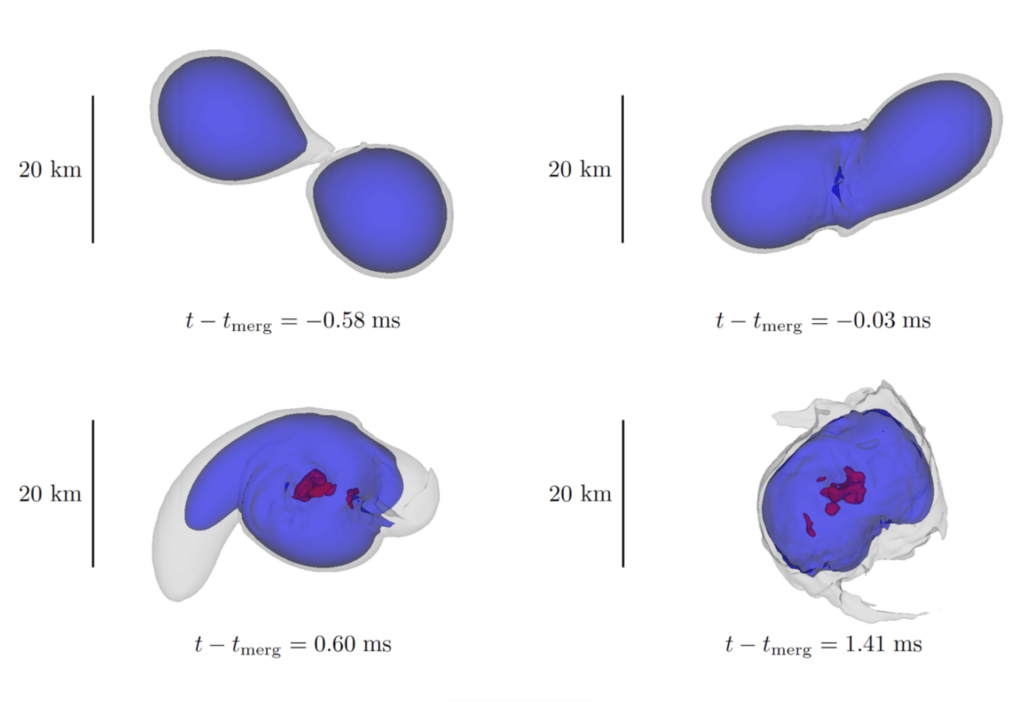


A long time ago in a galaxy far, far away, two neutron stars entered a collision course. Locked in an orbit around each other for millions of years, their separation became shorter as they revolved faster, radiating away part of their energies as gravitational waves. Eventually they collided and merged into a highly deformed hyper-massive neutron star which was accompanied by an intense explosion of matter and radiation called a kilonova.
Gravitational waves, which are ripples in the fabric of space-time caused by very fast motions of compact objects like neutron stars and black holes, were picked up from the above collision by the LIGO & VIRGO detectors on Earth on the 17th of August 2017. Shortly after that, a gamma ray burst from the same event was detected by several earth and space-based telescopes.
Neutron stars are one of the densest objects known to mankind. They pack matter of about 1.4 times the mass of the sun in about 12 miles which is roughly the size of downtown Manhattan. A tablespoon of neutron star material will weigh more than the Mt. Everest. When these stars collide, they generate extremely dense conditions that are several times the density of atomic nuclei and far from possible to replicate on earth. Therefore, observations of their mergers provide excellent test-beds of ultra-dense nuclear phenomena. Quantum Chromodynamics or QCD is the theory which explains the interactions of fundamental particles called quarks and gluons making up the protons and neutrons which in turn make up the nuclei and the matter around us. QCD states that quarks occur in confined states to make up protons and neutrons and interact via the strongest known force in nature called as the strong nuclear force. However, at ultra-high densities such as those produced during a neutron star merger, these confined quarks can melt into a soup of deconfined quark matter via a phase transition.
Our work attempts to calculate signatures of such a phase transition that may be imprinted on gravitational waves and electromagnetic waves like X-rays, ultraviolet or infrared. These waves can be detected by sensitive detectors like LIGO, swift, IceCube, etc. We use models developed by a team of nuclear theorists to simulate matter at ultra-high densities and use them as inputs to our general relativistic simulations of neutron star mergers. Whether or not quarks are produced in neutron star mergers, is an open question and hence we model two scenarios where quarks are produced vs. when they are not. This is shown in red and blue colours respectively in Figure 2 where the strain of the gravitational waves, as LIGO might detect them, is shown.
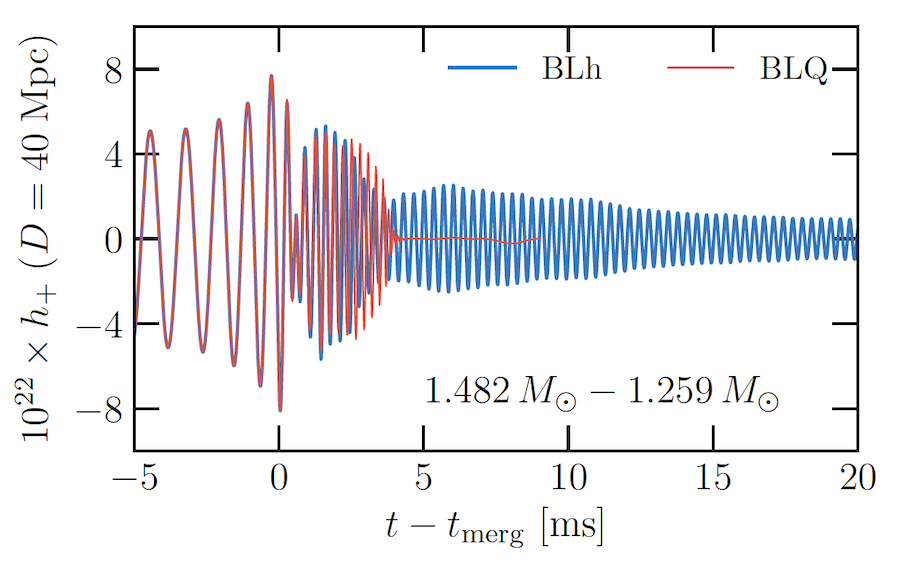
In Figure 2, we can observe that with an appearance of quarks, the gravitational wave signal in red dies out before the case when quarks are not produced i.e. the signal in blue. This early shutting off of gravitational waves is an indication that the deformed star after merger has collapsed into a black hole, a process that can be accelerated by the production of quarks.
In addition to gravitational waves, clues about the appearance of quarks in a merger of neutron stars may be imprinted on electromagnetic waves from the merger event which can be observed several months to years after the merger as synchrotron afterglows. Our study shows that a merger which produces quarks is expected to be slightly brighter in radio waves and X-rays, a long time after the merger, as compared to the ones where no quarks are produced.
Modelling neutron star mergers is one of the most challenging problems in physics requiring large computational resources and extensive mathematical modelling. They are also very subtle problems which combine all the 4 fundamental forces of nature: the strong force via quarks and gluons, the weak force via neutrinos, the electromagnetic force due to emission of radiation and extremely strong gravity. With the advent of multi-messenger astronomy, it has now become possible to study the universe through several windows of radiation be it electromagnetic, gravitational or neutrinos and a more cohesive picture of the behaviour of matter under extreme conditions has started to emerge.
This introduction was prepared by Aviral Prakash, N3AS Graduate Student and lead author of this research.
Read More: